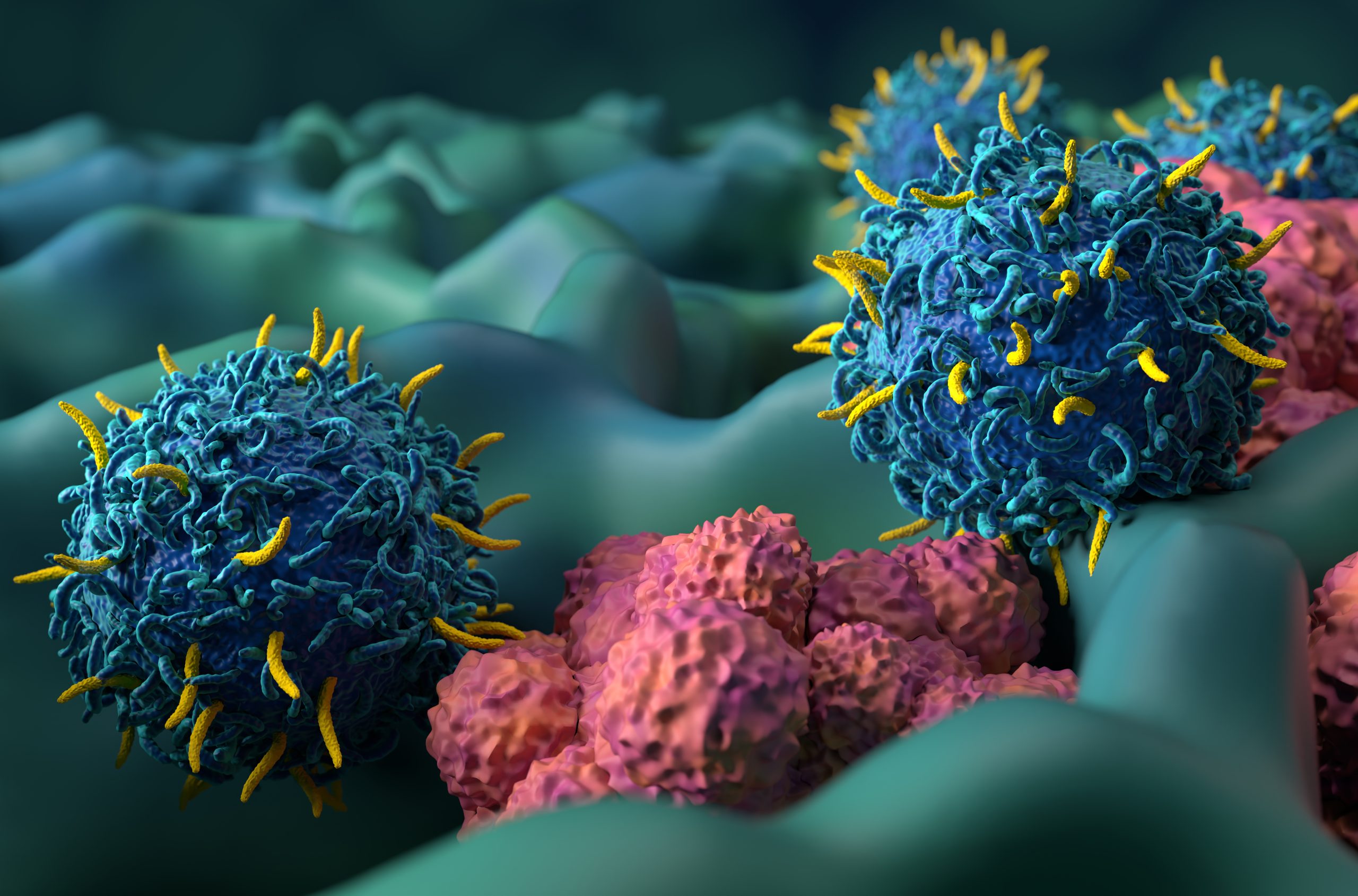
Technology review
Cancer cells can evade recognition by the immune system. One biotechnology aiming to address this is the use of chimeric antigen receptors (CARs). These are synthetic receptors that, when expressed in T cells, provide T cells with the ability to target a cognate ligand on a cancerous cell. Currently approved CAR-T cell therapies are manufactured by removing T cells from a patient, engineering them ex vivo,and then re-introducing them into the same patient. However, in vivo generation of CAR-T cells is an exciting prospect for this technology.
Extensive research has been performed to identify an optimal CAR structure that provides improved efficacy and safety. The current generation, the fifth-generation CARs, usually comprise an antigen-binding domain (to recognise the target antigen), a hinge domain (to provide length to the receptor for accessing the target antigen), a transmembrane domain (to anchor the CAR in the T cell membrane), an intracellular signalling domain (itself comprising a co-stimulation domain and a T cell activation domain), and an armour protein (such as an immunomodulatory protein) (Rafiq et al., 2019). This is in comparison to first-generation CARs, which do not have a co-stimulatory domain or an armour protein (Xin et al., 2022).
Despite the successes of CAR-T cell therapy, there are still ongoing technical challenges. Viral transduction is frequently used to deliver the CAR transgene to a T cell, as this method results in high transduction efficiency. However, some of the drawbacks of this delivery method are genotoxicity, small cargo capacity, and the risk of insertional mutagenesis, as the transgene can randomly integrate into the target DNA (Atsavapranee et al., 2021).
Gene editing tools, such as base editing, zinc-finger nucleases (ZFN), transcription activator-like effector nucleases (TALEN), and CRISPR-Cas9 allow for more precise editing of target DNA (Atsavapranee et al., 2021). These tools can be used to target and knock out candidate loci, for example, to allow for enhanced anti-tumour activity of T cells and/or to knock in the CAR construct at a particular locus of the T cell DNA.
Challenges also surround the efficacy of CAR-T cell therapy, as, for example, tumours may develop resistance to a single antigen. However, data using dual or tandem-targeted CAR constructs are showing promise. As target antigens may also be expressed on normal tissues, there is the risk of ’on-target off-tumour toxicity’. Research is investigating other targets, restricted to tumour cells, such as overexpressed post-translational modifications. Moreover, a solid tumour can act as a physical barrier, impacting the infiltration of CAR-T cells to the tumorigenic site and thereby affecting the efficacy of the treatment. To address this, research is studying the local administration of CAR-T cells and the expression of enzymes in CAR-T cells to degrade the tumour stroma. CAR-T cell therapy can also be limited by the tumour’s immunosuppressive microenvironment. Strategies to address this include altering the properties of CAR-T cells so that they are resistant to immunosuppression and promoting stimulation of immunostimulatory signals (Sterner and Sterner, 2021).
Administration of CAR-T cell therapy may give rise to adverse events characterised by toxicities such as cytokine-release syndrome, macrophage activation syndrome and immune effector cell-associated neurotoxicity syndrome. Strategies to prevent these from occurring include modifying the CAR structure, reducing CAR immunogenicity, and incorporating ’off-switches‘ – i.e. selectively eradicating the engineered T-cells upon an adverse event (Sterner and Sterner, 2021).
Finally, the process of manufacturing CAR-T cells is complicated, as extracting and engineering T cells from a patient requires significant time, expertise, and equipment, which can also make it extremely costly. New techniques to bypass this manufacturing process include the utilisation of universal CAR-T cell therapies, which use T cells from healthy donors instead of those from patients, thereby providing an ‘off-the-shelf’ product. However, this technique is complicated by graft-versus-host disease. Studies are shedding light on the in vivo generation of CAR-T cells, whereby nanoparticles and viral vectors comprising the CAR plasmid are introduced directly into the patient (Xin et al., 2022). In vivo generation may be the new future for CAR-T cell therapy.
Therefore, whilst CAR-T cell therapy has provided a promising treatment for cancer patients, further innovation is required to address its associated ongoing technical challenges.
Indications treated
To date, a total of six FDA- and EMA-approved CAR-T cell therapies are available. These include axicabtagene ciloleucel (Yescarta®), brexucabtagene autoleucel (Tecartus®), tisagenlecleucel (Kymriah®), and lisocabtagene maraleucel (Breyanzi®) for B cell lymphoma and leukaemia. Said therapies utilise an anti-CD19 antigen-binding domain (Cappell and Kochenderfer, 2023; Bellino et al., 2023). Idecabtagene vicleucel (Abecma®) and ciltacabtagene autoleucel (Carvykti®) are FDA- and EMA-approved for the treatment of relapsed and refractory multiple myeloma, and utilise either an anti-B cell maturation antigen (BCMA) or a dual anti-BCMA antigen-binding domain, respectively (Cappell and Kochenderfer, 2023; Bellino et al., 2023).
All of the above approved therapies use a second-generation CAR construct, meaning an antigen-binding domain, hinge domain, transmembrane domain, co-stimulatory domain, and a T cell activation domain. Furthermore, the above approved therapies utilise viral transduction to deliver the CAR gene to the T cell (Cappell and Kochenderfer, 2023).
Patent considerations
Due to the ongoing challenges associated with CAR-T cell therapy, there are exciting opportunities in the biotechnology sector to identify innovative solutions. It is important to take into consideration the intellectual property that may arise from research and development, and whether any patent applications could be filed to protect these valuable ideas.
Patent protection in this area includes claims to the CAR itself, polynucleotides encoding the CAR, vectors encoding said polynucleotides, immune cells comprising the CAR, compositions comprising said immune cells, and second medical use claims. Other claim categories include methods of manufacturing the engineered T cells.
Whilst patent protection provides a monopoly right for its owner, this does not give the owner the right to commercialise their product. Commercialising a product comes with the risk of infringing a patent. As the CAR-T cell therapy landscape is competitive, this has led to patent infringement disputes (Keown, 2019; Kanstein, 2024). Therefore, before commercialisation, it is advised to perform a freedom-to-operate search to assess whether the product could potentially infringe any existing and in-force patent(s).
Priority patent applications filed worldwide mentioning ‘chimeric antigen receptor’ saw a surge during the 2010s, which coincided with the timing of clinical trials.
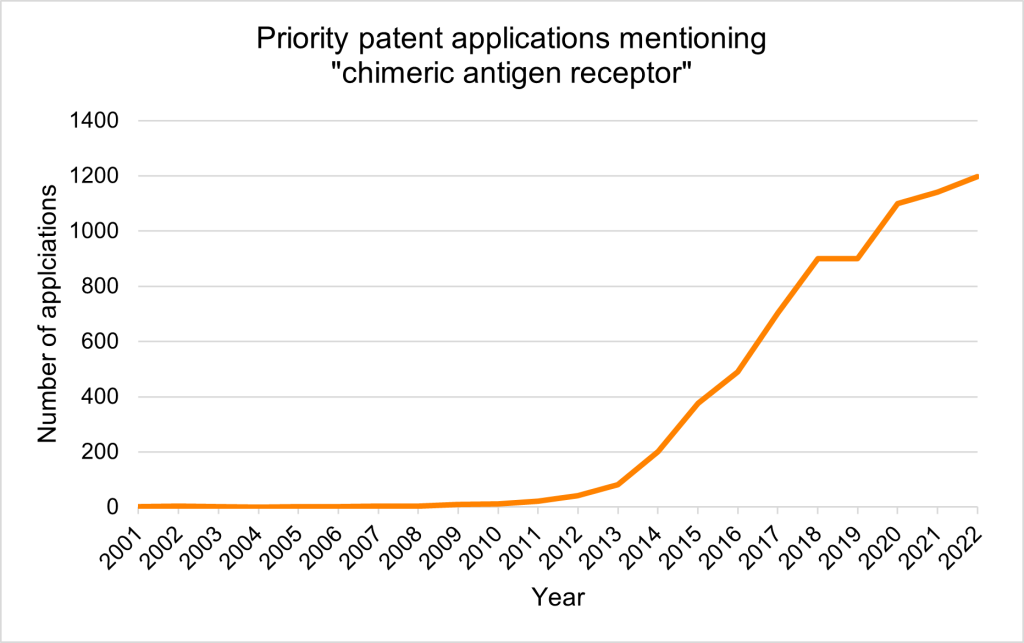
Given the structure of CARs, many structural combinations can arise based on the identity of each of the different domains of the receptor. Thus, one issue that may occur during search and examination is that a lack of unity objection is raised, where the European Patent Office (EPO) may consider the application to contain multiple inventions rather than a single invention, which is unallowable.
Furthermore, as CARs may include antigen-binding domains (e.g. scFvs or nanobodies), the EPO’s approach to patenting antibodies must be considered when prosecuting a patent application before the EPO (EPO GL G-II, 2).
During the search, the EPO will assess whether the invention as defined by the claims is novel and inventive, amongst other patentability requirements. To demonstrate an inventive step, it is important to consider the inclusion of data in the application, such as in vitro or in vivo data, which, for example, may show enhanced anti-tumorigenic effects. For example, in T 0203/22, claim 1 was found to be inventive based on data in the application as filed. The data showed that the claimed CAR, which exclusively targeted B7-H6, resulted in improved activation of T cells and anti-tumorigenic effects when compared to the closest prior art NKp30-based CAR (which was considered to have less specific targeting).
Supporting evidence for a therapeutic effect is also important from a sufficiency standpoint. In T 1502/22, the opponent argued that the medical use claims were not sufficiently disclosed, as there was no data to support a therapeutic effect for some of the cancers recited in said claims. However, in this decision, the Boards of Appeal were in favour of the patentee and considered the data in the application as filed, which showed activation and cytolytic activity of T cells expressing the claimed CAR, to be evidence of therapeutic activity.
Furthermore, it is also important to consider that under European practice, unlike for example, in the US, claims to a method of treatment are unallowable. However, substances or compositions for use in said methods of treatment are patentable. This consideration will be highly relevant for new innovations related to in vivo generation of CAR-T cells, which, as explained above, involves administering nanoparticles and viral vectors comprising the CAR plasmid directly into the patient. Given that different jurisdictions have different claim requirements for medical inventions, when drafting a patent application, care needs to be taken in providing the suitable basis for these claim categories should this need to be relied upon.
Summary
CARs and CAR-T cell therapy are exciting biotechnologies with many opportunities for further development and innovation. There are a variety of avenues to be explored in terms of modifying the structure of the CAR, delivery mechanisms of the CAR transgene, methods to improve the efficacy of the therapy and reduce any toxic side effects and improve the manufacturing of the engineered T cells. When looking to patent innovations in this area, consideration of the EPO’s patentability requirements is crucial.
The biotechnology group at GJE has extensive experience in patenting a diverse range of biotechnological inventions, in addition to advising innovative biotech companies and investors. To discuss your biotech IP strategy, please contact us at biotech@gje.com.
References
Alex Keown. 2019. Jury Awards BMS $752 Million in CAR-T Patent Infringement Case. Available online; accessed April 2025.
Atsavapranee, B. et al. 2021. ‘Delivery technologies for T cell gene editing: Applications in cancer immunotherapy’, EBioMedicine, vol. 67.
Bellino, S. et al. 2023. ‘Cell-based medicinal products approved in the European Union: current evidence and perspectives’, Front Pharmacol, vol. 14.
Cappell, K.M. and J.N. Kochenderfer. (2023) ‘Long-term outcomes following CAR T cell therapy: what we know so far’, Nat Rev Clin Oncol, vol. 20, no. 6, pp. 359-371.
Fraiser Kansteiner. 2024. Trump selects surgeon and author Martin Makary for FDA commissioner post. Available online; accessed April 2025.
Rafiq, S. et al. 2020. ‘Engineering strategies to overcome the current roadblocks in CAR T cell therapy’, Nat Rev Clin Oncol, vol. 17, pp. 147-167.
Sterner, R.C. and R.M. Sterner. 2021. ‘CAR-T cell therapy: current limitations and potential strategies’, Blood Cancer J, vol. 11.
Xin, Y. et al. 2022. ‘In-vivo induced CAR-T cell for the potential breakthrough to overcome the barriers of current CAR-T cell therapy’, Front Oncol, vol. 12.
E.P. Office, ‘Chapter II; 6. Antibodies’, in Guidelines for Examination in the European Patent Office, 2024.
European Patent Office. (2022). T 0203/22. Available online (Accessed: April 2025).
European Patent Office. (2022). T 1502/22. Available online (Accessed: April 2025).