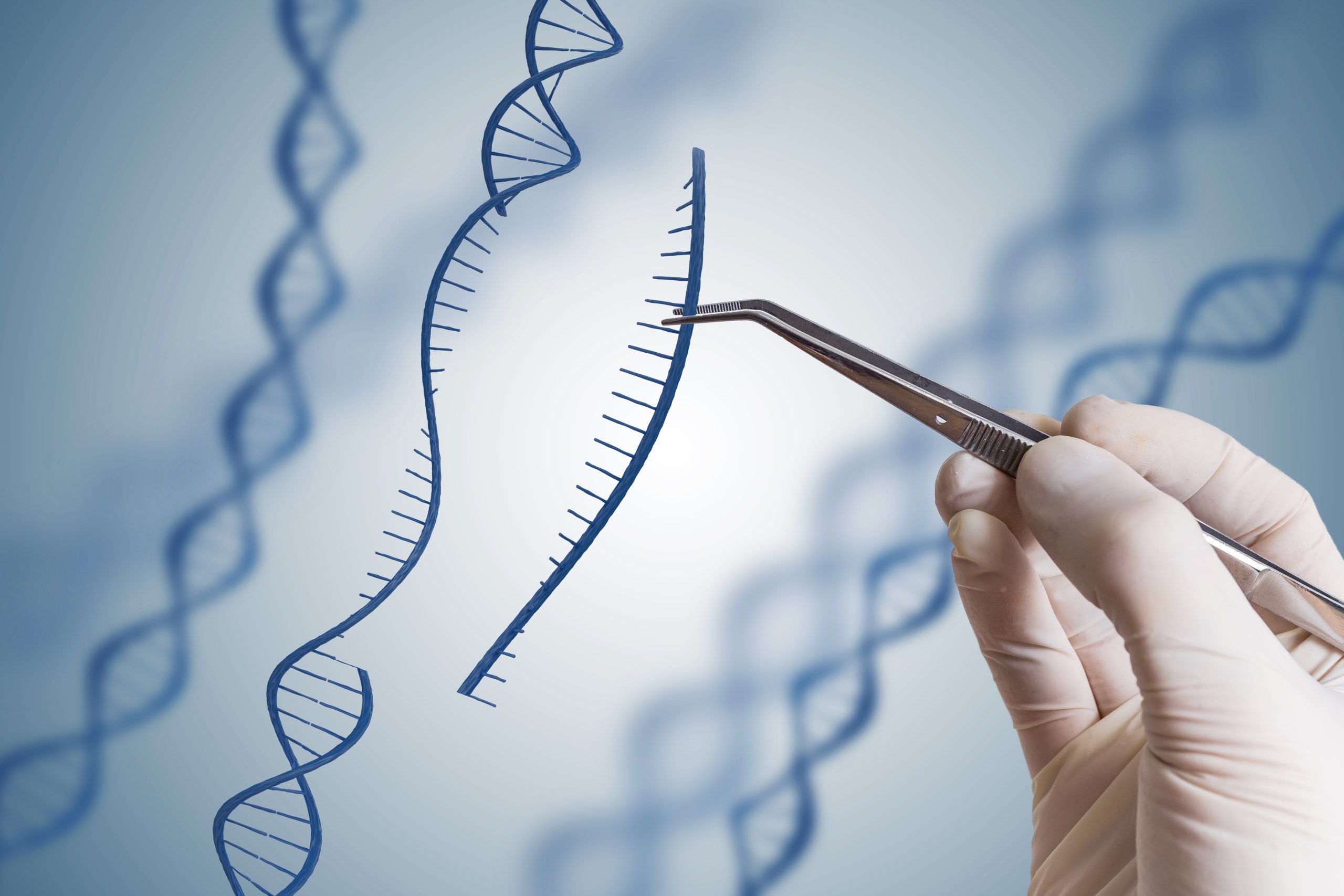
On 16 November 2023, the Medicines and Healthcare products Regulatory Agency (MHRA) in the UK approved the world’s first CRISPR-based gene therapy, which is also the first gene therapy for the treatment of sickle cell disease (SCD) and transfusion-dependent beta thalassemia (TDT) under the brand name CASGEVY™ (also known as ‘exa-cel’). CASGEVY™ is also under review by the Food and Drug Administration (FDA), the European Medicines Agency (EMA) and the Saudi Food and Drug Authority, with the FDA due to give a final decision on the approval of ‘exa-cel’ (formally known as CTX001™) for use in SCD and TDT on 8 December 2023.
CRISPR (an acronym for clustered regularly interspaced short palindromic repeats) is a family of DNA sequences found in the genomes of prokaryotic organisms, such as bacteria and archaea. Amazingly, in nature, these DNA sequences form the basis of a primitive immune system that bacteria use to identify and subsequently destroy bacteriophages (viruses that attack bacteria) that they have previously encountered. The CRISPR sequences include fragments of viral DNA to enable the bacteria to ‘remember’ past attacks.
However, it was only in 2012 that Professor Jennifer Doudna and Professor Emmanuelle Charpentier at the University of California, Berkeley, discovered that the CRISPR system, more specifically the CRISPR-Cas9 system, could be harnessed to make targeted, site-specific breaks and changes to the genome of prokaryotes (Jinek et al., 2012). Since then, CRISPR technology has allowed huge advances to be made in the gene-editing field, benefiting numerous industries. Indeed, Doudna and Charpentier were awarded the Nobel Laureate in Chemistry in 2020 for their outstanding contribution to science.
So, how does it actually work? The CRISPR-Cas system requires a CRISPR-associated (Cas) endonuclease (for example, Cas9 from Streptococcus pyogenes) to cleave the DNA and a single guide RNA (sgRNA). The sgRNA contains a sequence complementary to the target DNA and an anchor portion that together guide the Cas protein to the target sequence and holds it in place to enable DNA cleavage. A different Cas enzyme can be used to target different locations, depending on the sequences adjacent to the target. Effectively, these two components act as a pair of ‘molecular scissors’. Following cleavage of the DNA, the cell recognises that damage has occurred and attempts to repair it. By manipulating these repair mechanisms, new genetic information can be incorporated into the genome, or the site can be disrupted if repair is not required.
The ability of CRISPR-based therapy to easily edit specific sites within a given genome has bolstered the new frontier of curative medicine. We are now living in an age where diseases previously thought untreatable are, in theory, curable, potentially with a single dose of treatment. Biopharmaceutical companies are battling to get a foothold in this potentially very lucrative space.
CASGEVY™ is a CRISPR-based therapy targeted at patients suffering from SCD and TDT. SCD and TDT are two inherited blood disorders that affect red blood cells, specifically the oxygen-carrying protein haemoglobin. SCD results from an individual having two faulty copies of the β-globingene, leading to abnormalities in the haemoglobin protein and rigid, ‘sickle-shaped’ red blood cells that are less efficient at carrying oxygen and clump and block blood vessels, causing agonising pain and high risk of cardiac events and stroke. This results from a single base-pair point mutation in which the amino acid valine is substituted with glutamic acid in the resulting β-globin chain. TDT is also caused by various mutations of the β-globin gene, causing reduced production of the β-globin chain of the haemoglobin protein. At present, patients diagnosed with SCD and TDT require lifelong supportive treatment when episodes flare, including blood transfusion, with no effective therapies presently available. However, that could all be about to change with the MHRA approval of CASGEVY™ and the potential upcoming approval by the FDA (and possibly the EMA).
CASGEVY™ works by first removing and then modifying the patient’s own hematopoietic stem and progenitor cells (HSPCs) to enable them to produce red blood cells that have high levels of foetal haemoglobin (HbF). HbF is the form of the protein that is present during foetal development but following birth is no longer produced in favour of the adult form of haemoglobin. A key difference between HbF and adult haemoglobin is an enhanced affinity for oxygen. Indeed, HbF levels of 10–20% in adults have been shown to enhance survival rates and reduce vaso-occlusive complications in SCD patients (Tolu et al., 2019). It is known that the transcription factor, BCL11A, represses γ-globin expression (Liu et al., 2019) and therefore reduces the level of HbF. CASGEVY™ works by preventing the inhibitory effect of BCL11A by disrupting the erythroid-specific enhancer region of the BCL11A gene in CD34+ HSPCs, thus increasing the levels of HbF, which provides a protective effect in patients.
The MHRA approval of CASGEVY™ follows two global clinical trials in which the safety and efficacy of CASGEVY™ in the treatment of SCD and TDT was assessed. It has been reported that both trials met their respective primary outcome of patients becoming free from severe vaso-occlusive crises or transfusion independent for at least 12 months (see the full press release here). There are trade-offs for the patient as treatment requires the bone marrow to be depleted by undergoing chemotherapy prior to infusion of modified stem cells. However, most patients report that the benefits outweigh the risks and challenges inherent in the treatment. It is also worth noting that CASGEVY™ has been approved for only a subset of SCD and TDT patients. For example, eligible SCD patients must be 12 years or older, have recurrent vaso-occlusive crises, and have a certain genotype with no human leukocyte antigen-matched related hematopoietic stem cell donor available.
CASGEVY™ highlights the clinical potential of CRISPR-based therapies and the positive implications for patients. Indeed, the potential uses of CRISPR-based therapies are wide-reaching, with the current applications merely scratching the surface of what could be possible in the future. However, there are challenges to overcome that will be particular to each application of the technology. While patent wars rage around the technology’s early incarnations, innovators have many avenues to explore for generating new intellectual property as problems are solved. As with all medications, regulatory exclusivities go hand in hand with patent rights, and companies working in this space must ensure that a strong and future-focused intellectual property strategy is in place in order to effectively, and efficiently, capture and protect innovative developments. As this space gets more crowded, this is more important than ever before.
The biotech team at GJE have extensive experience in patenting nucleotide-based therapeutics. To discuss your biotech IP strategy, please find our contact details here and here or contact us at biotech@gje.com.
References
- Jinek M, Chylinski K, Fonfara I, Hauer M, Doudna JA, Charpentier E. A Programmable Dual-RNA-guided DNA Endonuclease in Adaptive Bacterial Immunity. Science. 2012 Aug 17;337(6096):816–21.
- Seda S. Tolu, Morayma Reyes Gil, Ugochi Olivia Ogu, Jui Choudhuri, Roger Fecher, Gracy Sebastian, Caterina P. Minniti. High Hemoglobin F in Sickle Cell Disease: Waning Protection with Age. Blood. 2019; 134 (Supplement_1): 3576.
- Liu N, Hargreaves VV, Zhu Q, Kurland JV, Hong J, Kim W, Sher F, Macias-Trevino C, Rogers JM, Kurita R, Nakamura Y, Yuan GC, Bauer DE, Xu J, Bulyk ML, Orkin SH. Direct Promoter Repression by BCL11A Controls the Fetal to Adult Hemoglobin Switch. Cell. 2018 Apr 5;173(2):430–442.e17.